Background
Central Nervous System (CNS) disorders are typically severe and may produce various behavioral, anatomical, and physiological changes in millions of patients, which complicates developing effective therapeutics and managing product-related neurotoxicity. Therefore, careful attention should be given to assessing potential neurotoxic findings that a therapeutic may induce and/or exacerbate. As part of the drug development process, health professionals should be mindful of the various technical approaches to evaluating drug-induced neurotoxicity; the challenges in assessing these hazards and characterizing them as risk based on the drug exposure levels each occurs at; and the future directions for CNS risk assessment.
CNS Risk Assessment Approaches
Drug-induced CNS risks may be evaluated via nonclinical pharmacokinetic (PK) and toxicokinetic, safety pharmacology, and toxicology studies typically required under the ICH M3 (R2) guidance (ICH M3 (R2) Guidance on Nonclinical Safety Studies for the Conduct of Human Clinical Trials and Marketing Authorization for Pharmaceuticals). This guidance is designed to assess various findings that include behavioral, physiological, and anatomical pathology data. Behavioral effects are assessed via the observation of clinical signs (e.g., convulsions and related premonitory signs), and alterations in simple (e.g., motor activity, sensory motor reflexes) and complex behaviors (e.g., learning and memory). Physiological effects are assessed via changes in electrical patterns in the brain (i.e., seizures), as well as alterations in visual and auditory examinations. Anatomical pathology within the CNS can be evaluated via various specialized stains (e.g., immunohistochemistry) to identify adverse microscopic findings in areas that include specific organelles, cells, and tissues. Across these approaches, technical and applied expertise is needed to design and conduct the related studies and to interpret the findings.
Challenges to Conducting CNS Risk Assessments
Conducting CNS risk assessments of drugs is a challenging process due to numerous target sites (e.g., ion channels, g-coupled receptors, enzymes) that may mediate the hazards observed, various levels of risk susceptibility across individuals, and the background levels of the CNS findings in the absence of treatment. See Table 1 for examples of FDA-approved drugs that induce CNS toxicities. The susceptibility to these and other drug-induced CNS hazards may be increased in organisms with a history of clinical and anatomical pathology findings (e.g., electrolyte imbalance; history of head injury) and physiological changes (marked decreases in cerebral blood flow), as well as in an environment with stressors (e.g., animals either restrained or presented with predators). These findings and others that occur spontaneously (i.e., background findings) may also complicate the interpretation and translation of CNS findings. For example, numerous publications have provided historical control data demonstrating the observation of convulsions/seizures in various animal species (Bielfelt et al., 1971; Buzsaki et al., 1990; Edmonds et al., 1979; Pearce et al., 2014; Satomoto et al., 2012). Together, these challenges provide insights into the issues that complicate conducting a risk assessment and the key factors that need to be considered when interpreting data, especially as part of determining whether a finding is drug-related or not.
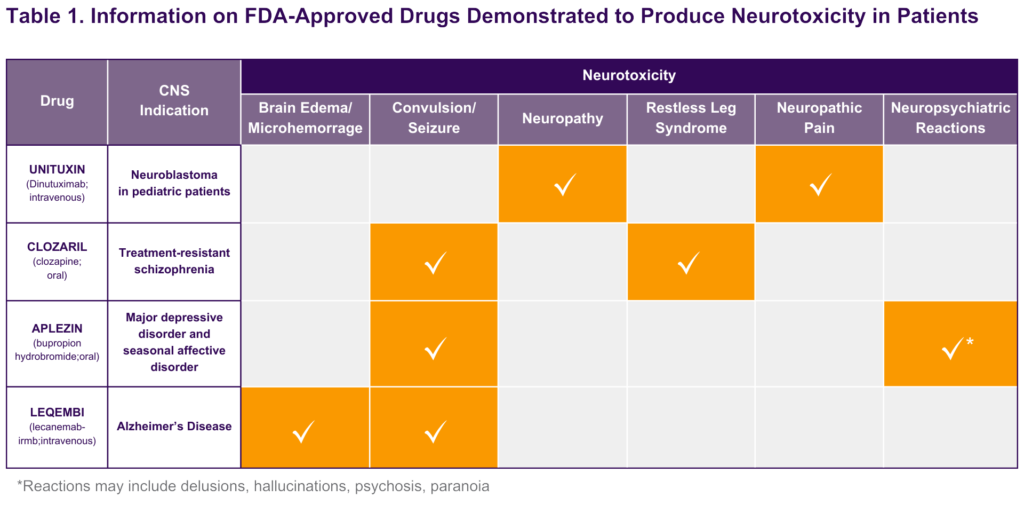
Approaches to Manage CNS Risks
CNS risks may be managed via various approaches including limiting exposure levels (i.e., setting adequate safety margins), excluding individuals with a history of brain injury or seizure, and employing safety monitoring (e.g., magnetic resonance imaging, electroencephalogram, self-report data). Given that there are no regulatory guidelines developed to help guide these approaches, each is typically done on a case-by-case basis. For example, drug-induced CNS risks such as seizures usually require a safety margin of 10X (i.e., maximum exposure allowed 10X lower than that at the No-Observed-Adverse-Effect-Level in the most sensitive species). In certain cases, the safety margin required may actually be > 10X, especially if the patient population is known to have an increased susceptibility to the expected risk (e.g., decreased seizure threshold). Managing CNS toxicities is a complicated process that must consider the physiological state, the drug history, and the many other factors that may impact the susceptibility of an individual to the potential drug-induced toxicities.
Future for CNS Risk Assessment
Given the severity of potential drug-induced CNS risks and the negative impact of these hazards on the development of therapeutic products, there is a significant need to improve the approaches used to characterize these risks. In particular, regulatory agencies have become increasingly interested in understanding and addressing age-related toxicities produced by drugs, as well as developing non-animal models for evaluating CNS toxicities. Concerns about age-related drug toxicities in the CNS may be associated with the development of the blood-brain barrier and the liver. The clinical relevance of these and related concerns is well established, which has led to the creation of pediatric requirements for drug development and other measures to ensure the safety of patients across age groups (for guidance see ICH S11 Guideline for Nonclinical Safety Testing in Support of Development of Paediatric Pharmaceuticals; and Guidance for Industry Nonclinical Safety Evaluation of Pediatric Drug Products). In regard to non-animal models, many have been developed to evaluate drug-induced CNS toxicities; however, the use of many of these models in supporting pivotal drug development decisions has been limited given concerns related to the clinical relevance of data collected and translating these findings to inform the design of clinical studies planned (for review see Bédard et al., 2020; Kim et al., 2020; Leung et al., 2022). For example, in vitro models that have been designed to evaluate potential drug-induced CNS hazards include organs-on-a-chip (e.g., for the blood-brain barrier, spinal cord, and nervous tissue) and brain organoids, which are designed to re-create the architecture and physiology of human organs (for review see Leung et al., 2022). Unlike these models, others that are more readily leveraged include PK and PD models. PK and PD models for CNS neurotherapeutics are frequently used to inform risk assessments and to support clinical decisions. For example, the comparative risk for a novel therapeutic with a clinically proven mechanism can be assessed by comparing in vitro findings with clinical drug exposure or PD related to therapeutic and adverse effects. Improvements to the approaches used to characterize CNS toxicities have been supported via the creation of guidance documents and new innovative models, which should help to ensure the safety of patients across age groups and the reduction of animals tested.
Bibliography
Bédard P, Gauvin S, Ferland K, Caneparo C, Pellerin È, Chabaud S and Bolduc S (2020) Innovative Human Three-Dimensional Tissue-Engineered Models as an Alternative to Animal Testing. Bioengineering 7:115.
Bielfelt SW, Redman HC and McClellan RO (1971) Sire- and sex-related differences in rates of epileptiform seizures in a purebred beagle dog colony. Am J Vet Res 32:2039-2048.
Buzsaki G, Laszlovszky I, Lajtha A and Vadasz C (1990) Spike-and-wave neocortical patterns in rats: genetic and aminergic control. Neuroscience 38:323-333.
Edmonds HL, Jr., Hegreberg GA, vanGelder NM, Sylvester DM, Clemmons RM and Chatburn CG (1979) Spontaneous convulsions in beagle dogs. Fed Proc 38:2424-2428.
Kim J, Koo BK and Knoblich JA (2020) Human organoids: model systems for human biology and medicine. Nat Rev Mol Cell Biol 21:571-584.
Leung CM, de Haan P, Ronaldson-Bouchard K, Kim G, Ko J, Rho HS, Chen Z, Habibovic P, Jeon NL, Takayama S, Shuler ML, Vunjak-Novakovic G, Frey O, Verpoorte E and Toh Y (2022) A guide to the organ-on-a-chip. Nature Reviews Methods Primers 2:34.
Pearce PS, Friedman D, Lafrancois JJ, Iyengar SS, Fenton AA, Maclusky NJ and Scharfman HE (2014) Spike-wave discharges in adult Sprague-Dawley rats and their implications for animal models of temporal lobe epilepsy. Epilepsy Behav 32:121-131.
Satomoto K, Kuroiwa Y, Masubuchi Y, Uemura H, Oshima Y and Okazaki S (2012) Spontaneous convulsions in Sprague-Dawley rats in carcinogenicity studies. J Toxicol Sci 37:645-647.