In our previous blog, Neurotoxic Risks in Therapeutics: Nonclinical Considerations (Part 1 of 2), we discussed how to utilize nonclinical information to detect and manage CNS toxicities such as seizures, convulsions, and CNS lesions, in drug development as a whole. In this follow-up blog, we narrow our focus to the specific clinical challenges (excluding abuse liabilities) in CNS-directed therapeutics and discuss how nonclinical and clinical data together inform the management of CNS toxicities in clinical studies.
CNS Therapeutics: Product Development Landscape
The availability of effective treatments for CNS disorders is a critical issue with global impact on human health. The World Health Organization estimates 1 in 4 people are affected by a neurological disorder, and there remains a large unmet need for CNS therapeutics. This need is expected to grow due to global increases in the incidence of CNS disorders as the human lifespan lengthens. With this imminent need for CNS therapeutics, well-informed drug development will play an essential role in providing safe and effective therapies to patients with neurological disorders.
Challenges to Develop CNS Therapeutics
Despite great demand for drugs that target the CNS, the drug development failure rate of these drugs is high. Successful drug development for CNS indications is hindered by the complexity of 1) the brain’s anatomy and physiological processes, and 2) etiology of CNS disorders. Several critical challenges which can lead to failure of a CNS drug candidate are described below:
- Difficulties Penetrating the CNS
- CNS-directed drugs must be able to cross the blood brain barrier (BBB) to reach the site of action and exhibit therapeutic activity. The BBB is made of cells that maintain homeostasis of brain parenchyma and cerebrospinal fluid (CSF). Due to the brain’s barriers, systemically or orally administered drugs often have a limited ability to enter the brain.
- There are a variety of methods that can be considered to promote brain entry, including designing drugs to have properties that will allow them into the CNS, and formulating alternate routes of administration such as intranasal and intrathecal.
- CNS-directed drugs must be able to cross the blood brain barrier (BBB) to reach the site of action and exhibit therapeutic activity. The BBB is made of cells that maintain homeostasis of brain parenchyma and cerebrospinal fluid (CSF). Due to the brain’s barriers, systemically or orally administered drugs often have a limited ability to enter the brain.
- Poor Efficacy
- Clinical failure rates of CNS drug candidates are higher than non-CNS drugs, often due to difficulties definitively demonstrating efficacy. These failures can be attributed to several different factors such as issues in delivering drug to the brain, excessive placebo effect, the inability of animal models to predict human efficacy, and poor pharmacokinetic (PK) exposure data.
- CSF is commonly used as a surrogate for assessing CNS exposure and can provide high quality PK data.
- Model-informed approaches can also be leveraged to predict CSF and brain exposure as well as to inform pharmacodynamic objectives in clinical studies.
- Clinical failure rates of CNS drug candidates are higher than non-CNS drugs, often due to difficulties definitively demonstrating efficacy. These failures can be attributed to several different factors such as issues in delivering drug to the brain, excessive placebo effect, the inability of animal models to predict human efficacy, and poor pharmacokinetic (PK) exposure data.
- Safety Concerns
- The CNS is central to our health and damage to the CNS can be life changing. Therefore, if a drug causes unacceptable neurotoxicity that cannot be mitigated, it will not be able to go forward in development. Unfortunately, many drugs including CNS-active drugs carry the risk of causing severe toxicities such seizures, convulsions, or both (Delatte 2019).
- When transitioning from dosing a drug in nonclinical studies to administering in humans, it is important to not only set proper safety margins (based on nonclinical data) for clinical exposure levels, but also have an adequate safety monitoring plan in place using modalities such as electroencephalograms (EEGs) and magnetic resonance imaging (MRI).
- The CNS is central to our health and damage to the CNS can be life changing. Therefore, if a drug causes unacceptable neurotoxicity that cannot be mitigated, it will not be able to go forward in development. Unfortunately, many drugs including CNS-active drugs carry the risk of causing severe toxicities such seizures, convulsions, or both (Delatte 2019).
In the next section, we will go into more detail about CNS-drug related toxicities (focusing on seizures) and how they may be managed in clinical studies.
CNS Risk Assessment: Management of Toxicities in Clinical Studies
In our approach to CNS risk management, we conduct a thorough evaluation of the available nonclinical information, including pharmacology, safety pharmacology, pharmacokinetics (PK), and toxicology data to create a detailed toxicity profile for the product tested. We also carefully consider available clinical PK data as well as other relevant data from original study reports or the public domain (see Table 1). Various types of data are used to confirm toxicities by understanding different aspects (e.g., functional, behavioral, and anatomical), as well as incidence and severity of the toxicities across multiple endpoints. By using an integrated approach, we ensure drug levels stay in an appropriate range and provide guidance on dose adjustment for treating patients who may have an increased vulnerability to drug-induced adverse events such as seizures due to their current physiological state.
- Analyzing Nonclinical Information
- Taking into account all nonclinical data in animals treated with a product allows for the identification of toxicities that may occur in humans.
- Identifying the maximum exposure levels for the drug based on the safety margins estimated in the most sensitive species tested.
- Designing Exclusion Criteria to Protect Vulnerable Subjects
- Excluding subjects with a history of head injury or seizures (if the study is not for a seizure indication) and those with levels of endogenous molecules outside normal ranges that are associated with seizure activity (Halawa et al 2011, Nardone et al 2016) helps to avoid harming subjects at higher risk for CNS adverse events. Understanding how neurological disease impacts the safety profile for a drug in a given patient population is important.
- Incorporating Real-time PK into Study Design
- Utilizing real-time PK assessments are instrumental in ensuring that no individual subject exceeds maximum exposure values (Cmax and AUC) prior to dose escalations. PK data are reviewed for each subject in the tested dose cohort prior to deciding if testing in the next cohort can be initiated. Sentinel subjects may also be used in dose escalation studies, with a review of PK and safety data prior to additional subjects being dosed.
The approach taken should be based on a risk/benefit analysis to ensure that the expected benefit(s) of the treatment exceed the potential risk(s) related to the product. By utilizing practices including the ones outlined above, Allucent is able to develop clinical protocols that both ensure the safety of the patients while addressing potential concerns of regulatory reviewers prior to engaging them.
Table 1. Examples of Drug-Induced Physiological States in Humans Impacting Seizure Susceptibility
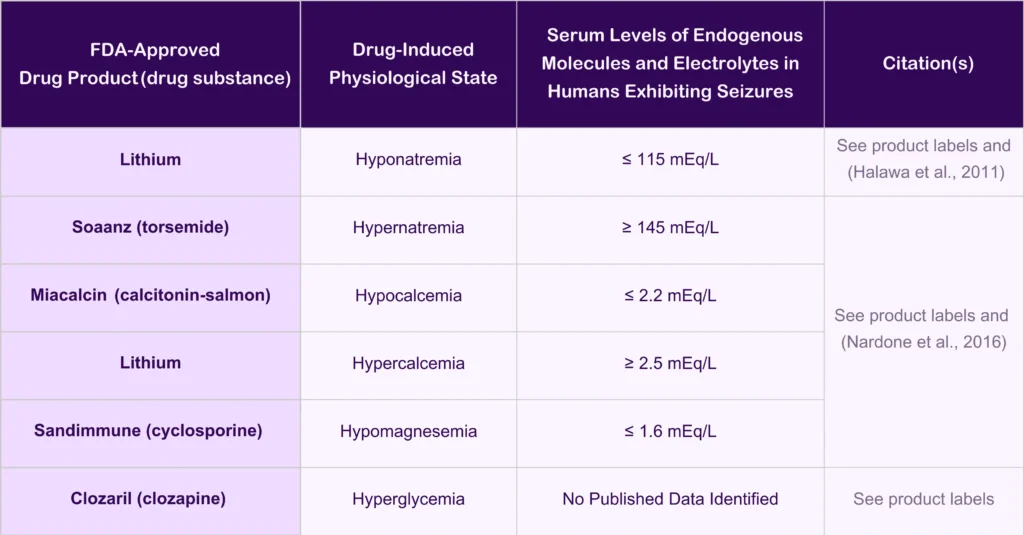
Lithium | Soaanz (Torsemide) | Miacalcin (Calcitonin-Salmon) | Sandimmune (Cyclosporine) | Clozaril (Clozapine)
Conclusions
In conclusion, navigating the complexities of the CNS drug development landscape requires an approach that integrates rigorous clinical and nonclinical data analysis, carefully considers patient physiology, and proactively employs risk mitigation strategies. Despite the challenges associated with CNS drug development, there is opportunity for incredible growth in this therapeutic space.
For a fuller understanding of CNS drugs and risk assessment, don’t miss Neurotoxic Risks in Therapeutics: Nonclinical Considerations (Part 1 of 2), which introduces the nonclinical foundations that complement the clinical insights discussed here. You can also explore the recent webinar Risk Assessment of Novel Neurotherapeutics: Mechanistic Translation of Preclinical Safety and PK/PD Data to a Clinical Setting featuring insights from the same expert team.
References
Delatte MS (2019) Issue Resolution Related to Convulsive Profiles in Advanced Issue Resolution in Safety Pharmacology (Mary Jeanne Kallman MKP ed) pp 203-223, Elsevier.
Halawa I, Andersson T and Tomson T (2011) Hyponatremia and risk of seizures: a retrospective cross-sectional study. Epilepsia 52:410-413.
Nardone R, Brigo F and Trinka E (2016) Acute Symptomatic Seizures Caused by Electrolyte Disturbances. J Clin Neurol 12:21-33.